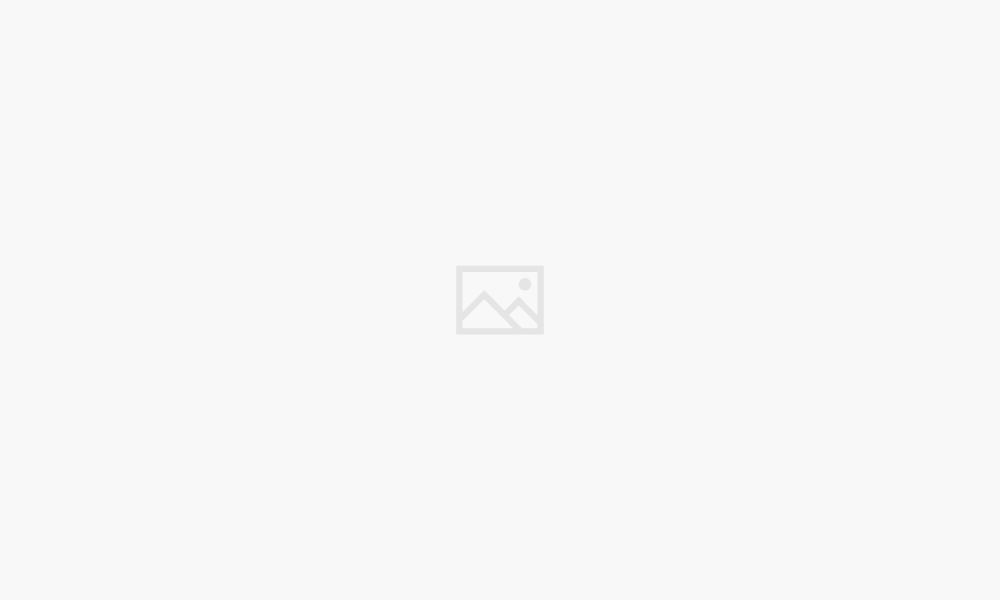
How do you determine rate law? This question lies at the heart of understanding chemical kinetics, the study of reaction rates. By unraveling the rate law, we gain insights into how reactions proceed, how fast they occur, and what factors influence their speed. This knowledge is crucial for various fields, from designing efficient industrial processes to understanding biological systems.
In essence, the rate law quantifies the relationship between a reaction’s rate and the concentrations of its reactants. It is expressed as an equation where the rate is proportional to the product of reactant concentrations raised to specific powers, known as the order of the reaction. The rate constant, denoted by ‘k’, is a proportionality constant that reflects the intrinsic speed of the reaction.
Introduction to Rate Laws
The study of chemical kinetics involves understanding how fast chemical reactions occur. Reaction rates, which quantify the speed of a chemical reaction, are essential for predicting the time required for a reaction to reach completion, optimizing reaction conditions, and designing new catalysts.
Reaction Rates and Their Measurement
Reaction rates are typically expressed as the change in concentration of a reactant or product over a specific time interval. The rate of a reaction can be measured by monitoring the change in concentration of a reactant or product over time.
- Spectrophotometry: This technique measures the absorbance or transmission of light through a solution, which can be related to the concentration of a species. The change in absorbance over time can be used to determine the rate of a reaction.
- Titration: This technique involves adding a reagent of known concentration to a solution containing the reactant until a specific endpoint is reached. The volume of reagent added can be used to determine the concentration of the reactant, and the change in concentration over time can be used to determine the rate of the reaction.
- Pressure Measurements: For reactions involving gases, the change in pressure over time can be used to determine the rate of the reaction. This is because the pressure of a gas is directly proportional to its concentration.
Rate Law and Its Components
The rate law is a mathematical expression that describes the relationship between the rate of a reaction and the concentrations of the reactants. It is expressed as:
Rate = k[A]m[B]n
Where:
- Rate: The rate of the reaction, typically expressed in units of concentration per unit time (e.g., M/s).
- k: The rate constant, which is a proportionality constant that reflects the intrinsic rate of the reaction at a given temperature. It is independent of the concentrations of the reactants.
- [A] and [B]: The concentrations of reactants A and B, respectively.
- m and n: The orders of the reaction with respect to reactants A and B, respectively. They are determined experimentally and represent the exponents to which the concentrations of each reactant are raised in the rate law.
Significance of Rate Laws in Understanding Reaction Mechanisms
Rate laws are not only useful for predicting reaction rates but also for gaining insights into the mechanism of a reaction. The mechanism of a reaction describes the step-by-step process by which reactants are transformed into products. The rate law can be used to determine the rate-determining step, which is the slowest step in the mechanism and therefore governs the overall rate of the reaction. By comparing the rate law with the proposed mechanism, chemists can gain valuable information about the elementary steps involved in the reaction and the nature of the transition states.
Experimental Methods for Determining Rate Laws
The rate law for a chemical reaction describes how the rate of the reaction depends on the concentrations of the reactants. There are several experimental methods that can be used to determine the rate law for a reaction. These methods involve measuring the rate of the reaction under different conditions and then analyzing the data to determine the order of the reaction with respect to each reactant.
Method of Initial Rates
The method of initial rates involves measuring the initial rate of a reaction at different initial concentrations of the reactants. The initial rate is the rate of the reaction at the very beginning of the reaction, before any significant amount of product has been formed. By comparing the initial rates at different concentrations, we can determine the order of the reaction with respect to each reactant.
To illustrate this method, consider the hypothetical reaction:
A + B → C
The rate law for this reaction can be written as:
Rate = k[A]^m[B]^n
Where:
– k is the rate constant
– [A] and [B] are the concentrations of reactants A and B, respectively
– m and n are the orders of the reaction with respect to A and B, respectively.
To determine the order of the reaction with respect to A, we can perform a series of experiments in which the initial concentration of A is varied while keeping the initial concentration of B constant. We then measure the initial rate of the reaction in each experiment. If doubling the initial concentration of A doubles the initial rate of the reaction, then the reaction is first order with respect to A (m = 1). If doubling the initial concentration of A quadruples the initial rate of the reaction, then the reaction is second order with respect to A (m = 2). In general, if the initial rate is proportional to [A]^m, then the reaction is mth order with respect to A.
Similarly, we can determine the order of the reaction with respect to B by varying the initial concentration of B while keeping the initial concentration of A constant.
Integrated Rate Law Method
The integrated rate law method involves deriving an equation that relates the concentration of a reactant to time. This equation is called the integrated rate law. The integrated rate law can be used to determine the rate constant and the order of the reaction.
The integrated rate law for a first-order reaction is:
ln[A]t – ln[A]0 = -kt
Where:
– [A]t is the concentration of reactant A at time t
– [A]0 is the initial concentration of reactant A
– k is the rate constant
The integrated rate law for a second-order reaction is:
1/[A]t – 1/[A]0 = kt
To determine the rate constant and order of a reaction using the integrated rate law method, we need to perform experiments in which we measure the concentration of a reactant at different times. We can then plot the data according to the integrated rate law for different orders of reaction. The plot that gives a straight line corresponds to the correct order of the reaction. The slope of the straight line is equal to the rate constant.
For example, if we plot ln[A]t versus time and obtain a straight line, then the reaction is first order. If we plot 1/[A]t versus time and obtain a straight line, then the reaction is second order.
Factors Affecting Reaction Rates
The rate of a chemical reaction can be influenced by several factors, including temperature, concentration, surface area, and the presence of catalysts. These factors affect the frequency of collisions between reactant molecules, the energy of these collisions, and the probability of successful collisions that lead to product formation.
Temperature
Temperature plays a crucial role in determining the rate of a chemical reaction. As temperature increases, the average kinetic energy of molecules increases, leading to more frequent and energetic collisions. This, in turn, increases the probability of molecules overcoming the activation energy barrier and reacting. The relationship between temperature and reaction rate is described by the Arrhenius equation:
k = Ae-Ea/RT
where:
* k is the rate constant
* A is the pre-exponential factor, which represents the frequency of collisions
* Ea is the activation energy
* R is the ideal gas constant
* T is the absolute temperature
The Arrhenius equation shows that the rate constant, and therefore the reaction rate, increases exponentially with temperature. For every 10°C increase in temperature, the reaction rate typically doubles. This is known as the rule of thumb.
Activation Energy, How do you determine rate law
Activation energy (Ea) is the minimum amount of energy that reactant molecules must possess in order to overcome the energy barrier and form products. The higher the activation energy, the slower the reaction rate. This is because fewer molecules will have enough energy to react at a given temperature.
- Reactions with high activation energies require a lot of energy to proceed, and therefore occur slowly.
- Reactions with low activation energies require less energy to proceed, and therefore occur more quickly.
Catalysts
Catalysts are substances that speed up the rate of a chemical reaction without being consumed in the process. They do this by providing an alternative reaction pathway with a lower activation energy. Catalysts do not change the equilibrium position of a reaction, they simply help the reaction reach equilibrium faster.
- Catalysts provide an alternative reaction pathway with a lower activation energy, increasing the rate of reaction.
- Catalysts are not consumed in the reaction, they are regenerated.
- Catalysts can be used to speed up or slow down reactions depending on the desired outcome.
Examples of Catalysts
- Enzymes are biological catalysts that speed up biochemical reactions in living organisms. For example, the enzyme lactase breaks down lactose, a sugar found in milk, into simpler sugars that can be absorbed by the body.
- Platinum is a catalyst used in the catalytic converter of cars to convert harmful pollutants like carbon monoxide and nitrogen oxides into less harmful gases like carbon dioxide and nitrogen.
- Catalysts are also used in the production of many important industrial chemicals, such as sulfuric acid, ammonia, and gasoline.
Applications of Rate Laws: How Do You Determine Rate Law
Rate laws are powerful tools that allow us to understand and predict the behavior of chemical reactions. They provide a framework for determining how the rate of a reaction changes under different conditions, such as temperature, concentration, or the presence of a catalyst. This knowledge is crucial for a wide range of applications, from designing efficient chemical processes to understanding the mechanisms of complex biological reactions.
Predicting Reaction Rates Under Different Conditions
Rate laws enable us to predict how the rate of a reaction will change when we alter the conditions. For example, we can determine how much faster a reaction will proceed if we increase the temperature or the concentration of reactants. This ability to predict reaction rates is essential for many applications, including:
* Optimizing chemical processes: By understanding how different factors affect reaction rates, chemists can optimize reaction conditions to maximize product yield and minimize waste. For example, by increasing the temperature, they can accelerate a reaction and achieve faster production.
* Designing new catalysts: Catalysts are substances that speed up chemical reactions without being consumed. Rate laws can be used to design new catalysts that are more efficient and selective, leading to cleaner and more sustainable chemical processes.
* Modeling complex systems: Rate laws are used to model complex systems, such as the atmosphere, the human body, and ecosystems. These models help us understand how these systems function and predict how they will respond to changes in conditions.
Designing and Optimizing Chemical Processes
Rate laws are fundamental to designing and optimizing chemical processes. By understanding how reaction rates are affected by different factors, engineers can design reactors and processes that maximize efficiency and minimize costs. Here are some examples:
* Reactor design: Rate laws help engineers design reactors that are optimized for specific reactions. For example, in the production of ammonia (NH3), a key component of fertilizers, the rate law for the Haber-Bosch process is used to determine the optimal reactor size and operating conditions.
* Process control: Rate laws are used to develop control systems that maintain the desired reaction rate and product quality. For example, in the production of pharmaceuticals, rate laws are used to ensure that the reactions proceed at the correct rate and that the desired product is formed.
* Process optimization: Rate laws can be used to optimize existing processes by identifying bottlenecks and finding ways to improve efficiency. For example, by understanding how the rate of a reaction is affected by temperature, engineers can optimize the operating temperature to maximize product yield and minimize energy consumption.
Real-World Applications
Rate laws find numerous applications in various fields:
* Pharmaceuticals: In the pharmaceutical industry, rate laws are used to study the degradation of drugs, determine their shelf life, and design drug delivery systems. Understanding the rate of drug degradation is crucial for ensuring the safety and effectiveness of medications.
* Environmental Science: Rate laws are essential for studying environmental processes, such as the degradation of pollutants in the environment, the formation of ozone in the atmosphere, and the cycling of nutrients in ecosystems. Understanding the rate of these processes is crucial for addressing environmental challenges.
* Materials Science: Rate laws are used to study the formation and properties of materials. For example, they can be used to predict the rate of corrosion of metals or the rate of polymerization of plastics. This knowledge is essential for designing durable and reliable materials.
Outcome Summary
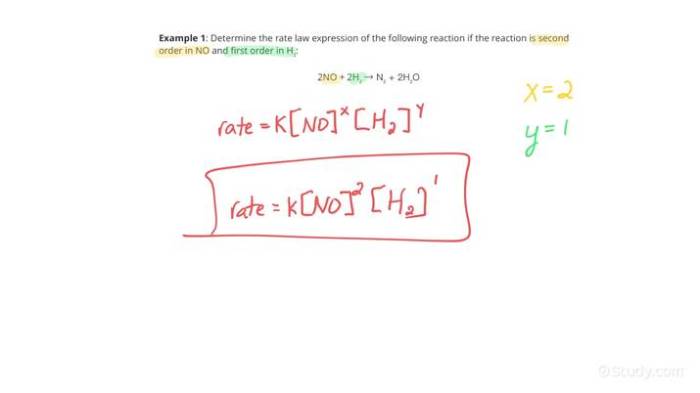
Determining rate laws is a cornerstone of chemical kinetics, allowing us to predict reaction rates under various conditions, optimize chemical processes, and understand the intricate mechanisms behind chemical transformations. By employing experimental methods like the initial rates method and integrated rate law analysis, we can decipher the secrets of how reactions unfold. This knowledge empowers us to manipulate and control chemical reactions, driving innovation in fields such as pharmaceuticals, environmental science, and materials science.
Q&A
What are some common units for the rate constant?
The units of the rate constant depend on the overall order of the reaction. For example, a first-order reaction has a rate constant with units of s-1, while a second-order reaction has a rate constant with units of M-1s-1.
How does temperature affect the rate constant?
The rate constant is highly temperature-dependent. As temperature increases, the rate constant typically increases exponentially, as described by the Arrhenius equation.
What is the difference between a rate law and a reaction mechanism?
A rate law describes the overall rate of a reaction, while a reaction mechanism provides a step-by-step description of how the reaction occurs. The rate law can be derived from the mechanism, but the mechanism cannot be determined solely from the rate law.