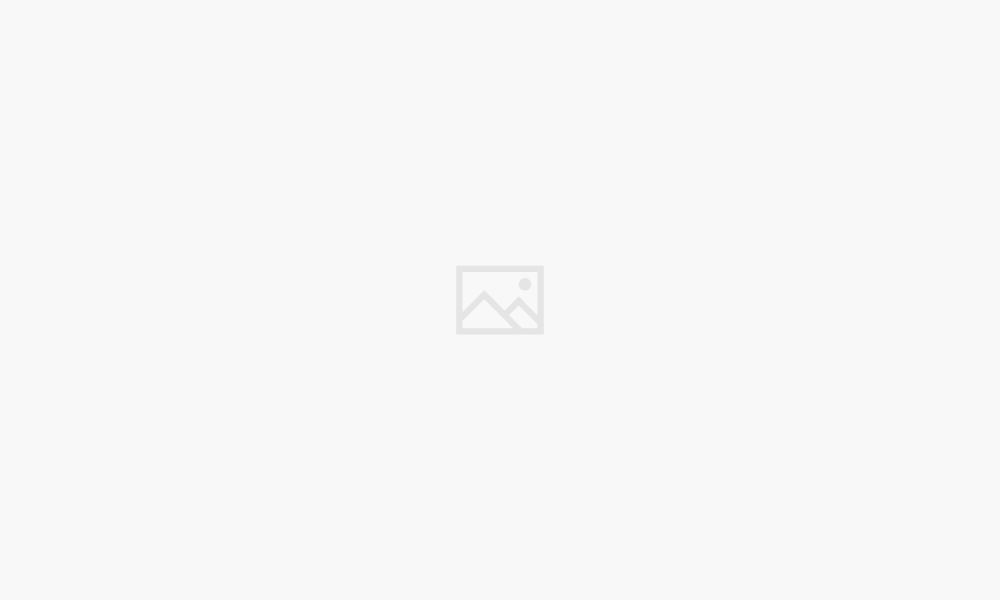
How many laws of thermodynamics are there? This question might seem simple, but it opens a door to a fascinating world of energy, heat, and the fundamental principles governing the universe. Thermodynamics, a branch of physics, deals with the relationships between heat, work, temperature, and energy. It’s a foundational science that applies to everything from the tiniest atoms to the largest stars.
The laws of thermodynamics are a set of fundamental principles that describe how energy behaves and interacts within physical systems. These laws are essential for understanding how engines work, how heat flows, and how energy can be transformed from one form to another. They provide a framework for comprehending the behavior of matter and energy in all their forms, from the smallest particles to the vast expanse of the cosmos.
Introduction to Thermodynamics
Thermodynamics is a fundamental branch of physics that deals with heat, work, temperature, and their relation to energy, radiation, and physical properties of matter. It is a cornerstone of many scientific disciplines, including chemistry, biology, and engineering, and plays a crucial role in understanding the behavior of systems at the macroscopic level.
Thermodynamics is essential for comprehending a wide range of phenomena, from the operation of engines and power plants to the functioning of living organisms. Its principles are applied in various fields, such as:
* Engineering: Designing efficient power plants, engines, and refrigeration systems.
* Chemistry: Predicting the feasibility and equilibrium of chemical reactions.
* Biology: Understanding energy flow in living organisms and biological processes.
* Meteorology: Studying atmospheric processes and weather patterns.
* Cosmology: Investigating the evolution of stars and the universe.
Energy and Its Forms
Energy is a fundamental concept in thermodynamics. It is defined as the capacity to do work. Work, in turn, is the transfer of energy that results in a change in the state of a system. Energy exists in various forms, including:
* Kinetic energy: The energy of motion, possessed by objects in motion.
* Potential energy: Stored energy due to an object’s position or configuration.
* Thermal energy: The internal energy of a system associated with the random motion of its constituent particles.
* Chemical energy: Energy stored in the bonds of molecules.
* Electrical energy: Energy associated with the flow of electric charges.
* Nuclear energy: Energy stored in the nucleus of an atom.
Thermodynamics in Everyday Phenomena
Thermodynamics governs many everyday phenomena, from the simple act of boiling water to the complex processes occurring in our bodies. Here are some examples:
* Boiling water: When water is heated, its thermal energy increases, causing its molecules to move faster and break free from the liquid state, transforming into steam.
* Refrigeration: Refrigerators work by using a refrigerant to absorb heat from the inside of the refrigerator and release it to the outside.
* Melting ice: Ice melts when it absorbs enough heat to overcome the forces holding its molecules in a rigid structure, transforming it into liquid water.
* Human metabolism: Our bodies use chemical energy from food to perform work, maintain body temperature, and carry out other life processes.
* Weather patterns: Atmospheric processes, such as the formation of clouds and precipitation, are driven by heat transfer and energy transformations.
The Zeroth Law of Thermodynamics
The Zeroth Law of Thermodynamics is a fundamental principle that establishes the concept of thermal equilibrium and forms the basis for temperature measurement. It was formulated after the first three laws of thermodynamics, hence its designation as the “Zeroth” Law.
Thermal Equilibrium
Thermal equilibrium is a state where two objects or systems in contact have the same temperature and no net heat transfer occurs between them. This means that the rate of energy transfer from one system to the other is equal in both directions.
“If two systems are each in thermal equilibrium with a third system, then they are in thermal equilibrium with each other.”
This statement forms the core of the Zeroth Law. It implies that if system A is in thermal equilibrium with system C, and system B is also in thermal equilibrium with system C, then system A and system B are also in thermal equilibrium with each other.
Temperature Measurement
The Zeroth Law enables temperature measurement by providing a framework for comparing the thermal states of different objects or systems.
To measure temperature, a thermometer is used as the “third system.” The thermometer is brought into contact with the object whose temperature is to be measured, and the thermometer’s temperature will change until it reaches thermal equilibrium with the object. The thermometer’s reading then provides a measure of the object’s temperature.
For example, a mercury thermometer works by expanding and contracting as its temperature changes. When the thermometer is placed in contact with an object, the mercury expands until it reaches thermal equilibrium with the object. The height of the mercury column in the thermometer then corresponds to the temperature of the object.
The First Law of Thermodynamics
The First Law of Thermodynamics is a fundamental principle in physics that describes the relationship between heat, work, and internal energy. It states that energy cannot be created or destroyed, only transferred or transformed from one form to another. This law is crucial for understanding how energy flows in various systems, from simple machines to complex biological processes.
Internal Energy, Heat, and Work
The First Law of Thermodynamics is expressed mathematically as:
ΔU = Q + W
Where:
* ΔU represents the change in internal energy of a system.
* Q represents the heat added to the system.
* W represents the work done by the system.
Internal energy (U) is the total energy contained within a system, including the kinetic energy of its molecules and the potential energy associated with their interactions. It is a state function, meaning its value depends only on the current state of the system, not on how it got there.
Heat (Q) is the transfer of thermal energy between objects at different temperatures. It is a process, not a state function, and can be positive (heat added to the system) or negative (heat removed from the system).
Work (W) is the energy transferred when a force acts over a distance. It can also be positive (work done by the system) or negative (work done on the system).
Examples of Energy Transformations and Conservation
The First Law of Thermodynamics is evident in various everyday phenomena:
* Burning fuel: When fuel burns, chemical energy is converted into heat and light energy. The total energy remains constant, but it is transformed from one form to another.
* Power plants: Power plants use heat from burning fuel to generate electricity. This process involves converting thermal energy into mechanical energy and then into electrical energy.
* Human body: Our bodies consume food to obtain chemical energy, which is then used for various functions, including movement, growth, and maintaining body temperature.
Implications of the First Law for Energy Efficiency
The First Law of Thermodynamics has significant implications for energy efficiency. It highlights the importance of minimizing energy losses during energy transformations. For example, in a power plant, some energy is always lost as heat, reducing the overall efficiency of the process.
The First Law also emphasizes that energy cannot be created from nothing. This principle is relevant in the context of renewable energy sources, where we seek to harness energy from natural sources like sunlight and wind. While these sources are renewable, the energy conversion processes involved are not always 100% efficient.
The Second Law of Thermodynamics
The Second Law of Thermodynamics is a fundamental principle in physics that governs the direction of energy flow and the tendency of systems to move towards a state of greater disorder. It is often stated in various ways, but the essence remains the same: energy cannot be perfectly converted from one form to another, and the entropy of an isolated system always increases.
Entropy and Its Implications
Entropy is a measure of disorder or randomness in a system. The more entropy a system has, the more disordered it is. The Second Law of Thermodynamics states that the entropy of an isolated system always increases over time. This means that systems naturally tend to move towards a state of greater disorder, unless external energy is supplied to maintain order.
The entropy of an isolated system never decreases.
Entropy has several important implications:
* Heat Flow: Heat always flows spontaneously from a hotter object to a colder object. This is because the hotter object has a higher entropy than the colder object, and heat transfer helps to equalize the entropy between the two objects.
* Spontaneous Processes: Many natural processes, such as the melting of ice or the diffusion of gases, are spontaneous because they lead to an increase in entropy.
* The Arrow of Time: The Second Law of Thermodynamics provides a directionality to time. It suggests that time moves forward, and processes cannot be reversed without external energy input.
Irreversibility of Natural Processes
The Second Law of Thermodynamics implies that natural processes are irreversible. This means that once a process has occurred, it cannot be reversed without external work being done. For example, if you drop a glass and it shatters, you cannot simply reverse the process to put the glass back together.
Examples of the Second Law in Energy Flow
The Second Law of Thermodynamics governs energy flow in many natural processes, including:
* Power Plants: Power plants convert heat energy into electrical energy. However, this conversion is not 100% efficient, and some energy is always lost as heat to the surroundings. This is a consequence of the Second Law, which states that some energy is always lost during energy transformations.
* Biological Systems: Living organisms are complex systems that require a constant supply of energy to maintain their order and function. They obtain this energy from food and use it to carry out various metabolic processes. However, these processes are not 100% efficient, and some energy is always lost as heat. This is why we generate body heat.
* The Universe: The Second Law of Thermodynamics applies to the entire universe. The universe is constantly expanding and becoming more disordered. This is because energy is being converted from one form to another, and some energy is always lost as heat.
The Third Law of Thermodynamics: How Many Laws Of Thermodynamics Are There
The Third Law of Thermodynamics, often referred to as Nernst’s theorem, provides a fundamental understanding of the behavior of matter at extremely low temperatures. It establishes a limit on the amount of entropy that a system can possess as its temperature approaches absolute zero.
Absolute Zero Temperature
Absolute zero, defined as 0 Kelvin (-273.15°C or -459.67°F), represents the theoretical point at which all molecular motion ceases. At this temperature, a system reaches its lowest possible energy state. While absolute zero is theoretically attainable, it is impossible to achieve in practice due to the limitations of cooling technologies and the quantum nature of matter.
Limitations of Reaching Absolute Zero
Reaching absolute zero is a challenging task due to several factors:
* Quantum Fluctuations: Even at extremely low temperatures, quantum fluctuations prevent the complete cessation of molecular motion. These fluctuations are inherent in the nature of quantum mechanics and ensure that some energy remains within the system.
* Cooling Technologies: Existing cooling technologies, such as refrigerators and cryostats, can achieve extremely low temperatures but cannot reach absolute zero. They rely on physical processes that have inherent limitations in achieving absolute zero.
* Heat Transfer: Even in a perfectly insulated system, there is always some heat transfer from the surroundings. This heat transfer prevents the system from reaching absolute zero.
Implications of the Third Law for Low-Temperature Phenomena, How many laws of thermodynamics are there
The Third Law of Thermodynamics has significant implications for low-temperature phenomena:
* Entropy at Absolute Zero: The Third Law states that the entropy of a perfect crystal at absolute zero is zero. This implies that the system is in a state of perfect order, with no randomness or disorder.
* Heat Capacity: As the temperature approaches absolute zero, the heat capacity of a system approaches zero. This means that it takes an increasingly smaller amount of energy to raise the temperature of the system.
* Superconductivity and Superfluidity: The Third Law provides a theoretical basis for the existence of superconductivity and superfluidity, phenomena observed at extremely low temperatures. These states are characterized by zero electrical resistance and zero viscosity, respectively.
* Quantum Phenomena: The Third Law is closely related to quantum mechanics and provides insights into the behavior of matter at the atomic and subatomic levels. It helps explain phenomena such as quantum tunneling and Bose-Einstein condensation.
Applications of Thermodynamics
Thermodynamics, a fundamental branch of physics, deals with heat and its relation to other forms of energy. Its principles govern energy transfer and transformation, making it applicable across various scientific and engineering disciplines.
Engineering
Thermodynamics plays a crucial role in various engineering fields, influencing the design and optimization of systems that involve energy transfer and transformation.
- Power Generation: Thermodynamics is the foundation for power plants, whether fueled by fossil fuels, nuclear energy, or renewable sources like solar and wind. The principles of heat transfer, work, and efficiency are used to design and analyze power generation systems, optimizing their performance and minimizing energy losses.
- Internal Combustion Engines: The operation of internal combustion engines, found in automobiles and other machinery, relies heavily on thermodynamic principles. The engine cycle, involving intake, compression, combustion, and exhaust, is analyzed using thermodynamic concepts to optimize fuel efficiency, power output, and minimize emissions.
- Refrigeration and Air Conditioning: Refrigeration and air conditioning systems rely on thermodynamic principles to transfer heat from one location to another. The principles of heat transfer, refrigeration cycles, and thermodynamic efficiency are crucial for designing and optimizing these systems.
Chemistry
Thermodynamics provides a framework for understanding and predicting chemical reactions and their equilibrium states.
- Chemical Equilibrium: Thermodynamic principles determine the equilibrium constant of a reaction, which indicates the relative amounts of reactants and products at equilibrium. This knowledge is essential for predicting the feasibility and direction of chemical reactions.
- Reaction Rates: While thermodynamics focuses on the energy changes in a reaction, it also influences reaction rates through the concept of activation energy. The activation energy, a thermodynamic quantity, determines the minimum energy required for a reaction to occur, influencing its rate.
- Phase Transitions: Thermodynamics explains the behavior of matter during phase transitions, such as melting, freezing, boiling, and condensation. The enthalpy and entropy changes associated with these transitions are determined by thermodynamic principles, allowing for predictions and control of these processes.
Biology
Thermodynamics is essential for understanding biological processes, from the molecular level to the functioning of entire organisms.
- Metabolism: Living organisms constantly exchange energy with their surroundings through metabolic processes. Thermodynamics explains the energy flow within cells, the efficiency of energy conversion, and the regulation of metabolic pathways.
- Enzyme Kinetics: Enzymes, biological catalysts, accelerate chemical reactions within cells. Thermodynamic principles are used to understand enzyme kinetics, including the activation energy required for enzyme-catalyzed reactions and the factors affecting their efficiency.
- Ecosystems: Thermodynamics applies to the flow of energy through ecosystems. The principles of energy transfer, efficiency, and entropy are used to understand the interactions between organisms and their environment, including the cycling of nutrients and the stability of ecosystems.
Meteorology
Thermodynamics is crucial for understanding weather patterns and climate change.
- Atmospheric Circulation: The movement of air masses in the atmosphere is driven by differences in temperature and pressure, which are governed by thermodynamic principles. The principles of heat transfer, convection, and atmospheric stability are essential for understanding and predicting weather patterns.
- Climate Modeling: Thermodynamics is used in climate models to simulate the Earth’s climate system. These models incorporate thermodynamic principles to account for energy transfer, heat absorption and release, and the effects of greenhouse gases on global temperatures.
- Cloud Formation: The formation of clouds is a thermodynamic process involving the condensation of water vapor. The principles of heat transfer, saturation, and latent heat are used to understand cloud formation and its role in weather patterns.
Cosmology
Thermodynamics plays a significant role in understanding the evolution of the universe.
- Big Bang Cosmology: The Big Bang theory, the prevailing model for the origin and evolution of the universe, relies on thermodynamic principles. The early universe is described as a hot, dense state, and its subsequent expansion and cooling are governed by the laws of thermodynamics.
- Black Hole Thermodynamics: Black holes, massive objects with extreme gravity, are also subject to thermodynamic principles. The concept of black hole entropy, a measure of the information lost in a black hole, is a fascinating area of research in cosmology.
- Cosmic Microwave Background Radiation: The cosmic microwave background radiation, a faint afterglow of the Big Bang, provides evidence for the universe’s initial hot state. The study of this radiation involves thermodynamic principles, including the laws of blackbody radiation and the concept of thermal equilibrium.
Beyond the Traditional Laws
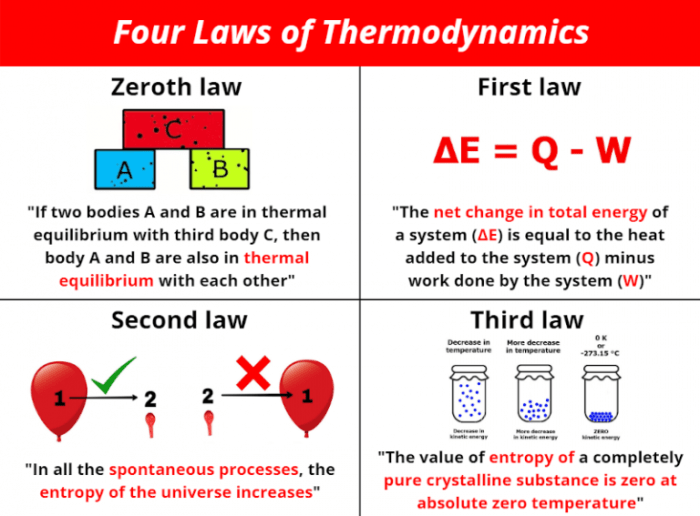
The traditional laws of thermodynamics, while foundational, have limitations in describing certain complex systems and phenomena. Modern research has expanded the field to encompass new ideas and approaches that provide a more comprehensive understanding of energy and its transformations. These emerging concepts offer valuable insights into the behavior of systems far from equilibrium, the role of information in thermodynamics, and the fundamental nature of entropy.
Non-Equilibrium Thermodynamics
Non-equilibrium thermodynamics deals with systems that are not in a state of thermodynamic equilibrium, meaning they are constantly exchanging energy and matter with their surroundings. This branch of thermodynamics explores the dynamics of these systems and the irreversible processes that govern their behavior.
- Irreversible Processes: In contrast to reversible processes, which are idealized and theoretically possible, irreversible processes are the reality of most natural systems. These processes involve energy dissipation and entropy production, leading to a gradual approach towards equilibrium.
- Fluctuation Theorems: These theorems quantify the probability of observing fluctuations in a system’s energy and entropy, even when it is in a steady state. They provide a statistical framework for understanding the dynamics of non-equilibrium systems and their response to external forces.
- Applications: Non-equilibrium thermodynamics finds applications in various fields, including:
- Biology: Understanding energy flow in living organisms, such as cellular processes and metabolic pathways.
- Materials Science: Designing materials with specific properties, such as heat conductivity and thermal stability.
- Climate Science: Analyzing the energy balance of the Earth’s atmosphere and predicting climate change.
Statistical Mechanics
Statistical mechanics bridges the gap between microscopic and macroscopic descriptions of thermodynamic systems. It uses probabilistic methods to explain the behavior of large ensembles of particles, providing a deeper understanding of the origins of thermodynamic laws.
- Ensemble Theory: Statistical mechanics uses the concept of ensembles, which are hypothetical collections of identical systems in different microstates. By analyzing the statistical properties of these ensembles, it is possible to derive thermodynamic quantities such as temperature, pressure, and entropy.
- Fluctuations and Correlations: Statistical mechanics allows for the study of fluctuations in thermodynamic quantities and the correlations between different parts of a system. This understanding is crucial for understanding the behavior of complex systems and for developing new materials and technologies.
- Applications: Statistical mechanics finds applications in:
- Solid-State Physics: Understanding the properties of materials, such as their electrical conductivity and magnetic behavior.
- Chemical Kinetics: Describing the rates and mechanisms of chemical reactions.
- Cosmology: Studying the evolution of the universe and the behavior of matter at high densities and temperatures.
Information Thermodynamics
Information thermodynamics explores the relationship between information, entropy, and energy. It recognizes that information can have a thermodynamic value and can influence the behavior of physical systems.
- Landauer’s Principle: This principle states that erasing one bit of information requires a minimum amount of energy dissipation, quantifying the thermodynamic cost of information processing.
- Maxwell’s Demon: This thought experiment highlights the role of information in thermodynamics. A hypothetical demon could seemingly violate the second law of thermodynamics by using information to separate hot and cold particles, but this requires energy expenditure to acquire and process the information.
- Applications: Information thermodynamics has potential applications in:
- Nano-scale Systems: Understanding the thermodynamic limits of information processing in nanoscale devices.
- Quantum Information: Exploring the relationship between information and entropy in quantum systems.
- Artificial Intelligence: Developing more efficient algorithms for machine learning and artificial intelligence by considering the thermodynamic cost of information processing.
Final Thoughts
The laws of thermodynamics are not merely abstract concepts; they have profound implications for our daily lives and technological advancements. They guide the development of power plants, refrigerators, and even the design of materials. Understanding these laws empowers us to harness energy efficiently, minimize waste, and unlock new possibilities in science and engineering. As we delve deeper into the intricacies of thermodynamics, we uncover a universe of interconnected phenomena, where energy reigns supreme and the laws of nature dictate the flow of everything around us.
Popular Questions
What is the difference between heat and temperature?
Heat is the transfer of thermal energy between objects at different temperatures. Temperature, on the other hand, is a measure of the average kinetic energy of the particles within an object.
Why is the Zeroth Law of Thermodynamics important?
The Zeroth Law establishes the concept of thermal equilibrium, which is crucial for defining and measuring temperature. It provides a basis for comparing the temperatures of different objects.
What is the significance of entropy?
Entropy is a measure of disorder or randomness in a system. The Second Law of Thermodynamics states that entropy always increases in an isolated system, implying that processes tend to move towards greater disorder.
Can we ever reach absolute zero temperature?
The Third Law of Thermodynamics states that absolute zero is unattainable, meaning we can get arbitrarily close to it but never reach it. This is due to the quantum nature of matter and energy.